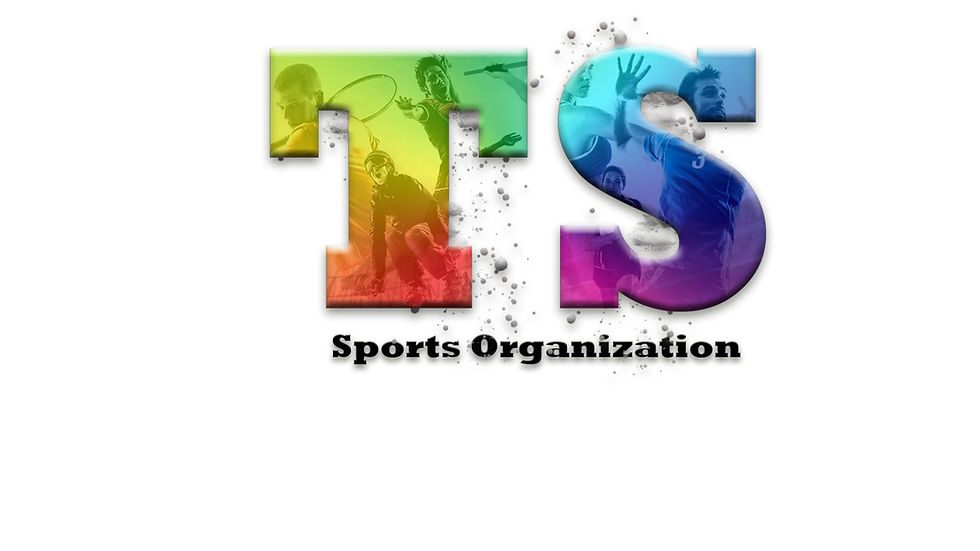
The process by which the body breaks down substrates with the aid of oxygen to generate energy is called cellular respiration. Because oxygen is required, this is an aerobic process. Unlike the anaerobic production of ATP that occurs in the cytoplasm of the cell, the oxidative production of ATP occurs within special cell organelles called mitochondria. In muscles, these are adjacent to the myofibrils and are also scattered throughout the sarcoplasm. Muscles need a steady supply of energy to continuously produce the force needed during long-term activity. Unlike anaerobic ATP production, the oxidative system is slow to turn on; but it has a much larger energy-producing capacity, so aerobic metabolism is the primary method of energy production during endurance activities. This places considerable demands on the cardiovascular and respiratory systems to deliver oxygen to the active muscles. Oxidative energy production can come from carbohydrates (starting with glycolysis) or fats. Oxidation of Carbohydrate. Oxidative production of ATP from carbohydrates involves three processes:
· Glycolysis
· The Krebs cycle
· The electron transport chain
Glycolysis In carbohydrate metabolism, glycolysis plays a role in both anaerobic and aerobic ATP production. The process of glycolysis is the same regardless of whether oxygen is present. The presence of oxygen determines only the fate of the end product, pyruvic acid. Recall that anaerobic glycolysis produces lactic acid and only three net mol of ATP per mol of glycogen, or two net mol of ATP per mol of glucose. In the presence
of oxygen, however, the pyruvic acid is converted into a compound called acetyl coenzyme A (acetyl CoA). Krebs Cycle Once formed, acetyl CoA enters the Krebs cycle (also called the citric acid cycle or tricyclic acid cycle), a complex series of chemical reactions that permit the complete oxidation of acetyl CoA. Recall that, for every glucose molecule that enters the glycolytic pathway, two molecules of pyruvate are formed. Therefore, each glucose molecule that begins the energy-producing process in the presence of oxygen results in two complete Krebs cycles. The conversion of succinyl CoA to succinate in the Krebs cycle results in the generation of guanosine triphosphate or GTP, a high-energy compound similar to ATP. GTP then transfers a Pi to ADP to form ATP. These two ATPs (per molecule of glucose) are formed by substrate-level phosphorylation. So at the end of the Krebs cycle, two additional mol of ATP have been formed directly, and the original carbohydrate has been broken down into carbon dioxide and hydrogen. Like the other pathways involved in energy metabolism, Krebs cycle enzymes are regulated by negative feedback at several steps in the cycle. The rate-limiting enzyme in the Krebs cycle is isocitrate dehydrogenase, which, like PFK, is inhibited by ATP and activated by ADP and Pi, as is the electron transport chain. Because muscle contraction relies on the availability of calcium in the cell, excess calcium also stimulates the ratelimiting enzyme isocitrate dehydrogenase.
Electron Transport Chain During glycolysis, hydrogen ions are released when glucose is metabolized to pyruvic acid. Additional hydrogen ions are released
in the conversion of pyruvate to acetyl CoA and at several steps in the Krebs cycle. If these hydrogen ions remained in the system, the inside of the cell would become too acidic. What happens to this hydrogen?
The Krebs cycle is coupled to a series of reactions known as the electron transport chain. The hydrogen ions released during glycolysis, during the conversion of pyruvic acid to acetyl CoA, and during the Krebs cycle combine with two coenzymes: nicotinamide adenine dinucleotide (NAD) and flavin adenine dinucleotide (FAD), converting each to its reduced form (NADH and FADH2, respectively). During each Krebs cycle, three molecules of NADH and one molecule of FADH2 are produced. These carry the hydrogen atoms (electrons) to the electron transport chain, a group of mitochondrial protein complexes located in the inner mitochondrial membrane. These protein complexes contain a series of enzymes and iron-containing proteins known as cytochromes. As high-energy electrons are passed from complex to complex along this chain, some of the energy released by those reactions is used to pump H+ from the mitochondrial matrix into the outer mitochondrial compartment. As these hydrogen ions move back across the membrane down their concentration gradient, energy is transferred to ADP, and ATP is formed. This final step requires an enzyme known as ATP synthase. At the end of the chain, the H+ combines with oxygen to form water, thus preventing acidification of the cell. This is illustrated in figure 2.10. Because this overall process relies on oxygen as the final acceptor of electrons and H+, it is referred to as oxidative phosphorylation.
For every pair of electrons transported to the electron transport chain by NADH, three molecules of ATP are formed, while the electrons passed through the electron transport chain by FADH yield only two molecules of ATP. However, because the NADH and FADH are outside the membrane of the mitochondria, the H+ must be shuttled through the membrane, which requires energy to be utilized. So in reality, the net yields are only 2.5 ATPs per NADH and 1.5 ATPs per FADH. Energy Yield From Oxidation of Carbohydrate The complete oxidation of glucose can generate 32 molecules of ATP, while 33 ATPs are produced from one molecule of muscle glycogen. The sites of ATP production are summarized in figure 2.11. The net production of ATP from substrate-level phosphorylation in the glycolytic pathway leading into the Krebs cycle results in a net gain of two ATPs (or three from glycogen). A total of 10 NADH molecules leading into the electron transport chain—two in glycolysis, two in the conversion of pyruvic acid to acetyl CoA, and six in the Krebs cycle—yields 25 net ATP molecules.
Remember that while 30 ATPs are actually produced, the energy cost of transporting ATP across membranes uses five of those ATPs. The two FAD molecules in the Krebs cycle that are involved in electron transport result in three additional net ATPs. And finally, substrate-level phosphorylation within the Krebs cycle involving the molecule GTP adds another two ATP molecules. Accounting for the energy cost of shuttling electrons across the mitochondrial membrane is a relatively new concept in exercise physiology, and many textbooks still refer to net energy productions of 36-39 ATPs per molecule of glucose.
Oxidation of Fat
As noted earlier, fat also contributes importantly to muscles’ energy needs. Muscle and liver glycogen stores can provide only ~2,500 kcal of energy, but the fat stored inside muscle fibers and in fat cells can supply at least 70,000 to 75,000 kcal, even in a lean adult. Although many chemical compounds (such as triglycerides, phospholipids, and cholesterol) are classified as fats, only triglycerides are major energy sources. Triglycerides are stored in fat cells and between and within skeletal muscle fibers. To be used for energy, a triglyceride must be broken down to its basic units: one molecule of glycerol and three FFA molecules. This process is called lipolysis, and it is carried out by enzymes known as lipases.
Free fatty acids are the primary energy source for fat metabolism. Once liberated from glycerol, FFAs can enter the blood and be transported throughout the body, entering muscle fibers by either simple diffusion or transporter-mediated (facilitated) diffusion. Their rate of entry into the muscle fibers depends on the concentration gradient. Increasing the concentration of FFAs in the blood increases the rate of their transport into muscle fibers. b-Oxidation Recall that fats are stored in the body in two places, within muscle fibers and in adipose tissue cells called adipocytes. The storage form of fats is triglyceride, which is broken down into FFAs and glycerol for energy metabolism. Before FFAs can be used for energy production, they must be converted to acetyl CoA in the mitochondria, a process called b-oxidation. Acetyl CoA is the common intermediate through which all substrates enter the Krebs cycle for oxidative metabolism. b-Oxidation is a series of steps in which two-carbon acyl units are chopped off of the carbon chain of the FFA. The number of steps depends on the number of carbons in the FFA, usually between 14 and 24 carbons. For example, if an FFA originally has a 16-carbon chain, b-oxidation yields eight molecules of acetyl CoA. The acyl units become acetyl CoA, which then enters the Krebs cycle for the formation of ATP.
On entering the muscle fiber, FFAs must be enzymatically activated with energy from ATP, preparing them for catabolism (breakdown) within the mitochondria. Like glycolysis, b-oxidation requires an input energy of two ATPs for activation but, unlike glycolysis, produces no ATPs directly.
References:
Kenney L.W., Wilmore J.H., Costill D.L. "Physiology of Sport and Exercise"
Comments